University certificate
The world's largest faculty of medicine”
Why study at TECH?
Access the latest developments in this field thanks to this update program and learn more about the use of software for biomedical signal processing"
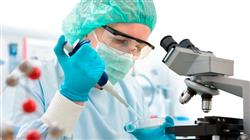
The integration of new technological tools in the biomedical field has led to rapid progress in this discipline. For this reason, in recent years, biomedical engineering has emerged as one of the most cutting-edge fields of healthcare, as it incorporates the most promising scientific advances to respond to a whole series of current medical challenges. Therefore, the specialist needs access to an up-to-date program such as this one to keep abreast of the latest developments in this field.
This Professional master’s degree in Biomedical Engineering delves into innovations and in areas such as biodevices and biosensors, fluid mechanics within the field of biomechanics, nanoparticles, metallic biomaterials, computed tomography, the application of artificial intelligence through the field of artificial vision to the medical field or the use of databases, among many others.
All this, following a 100% online learning methodology that allows the professional to choose the time and place to study, since it adapts to their personal circumstances. In addition, a high-level teaching staff specialized in biomedical engineering will guide the physician using numerous multimedia teaching resources such as video procedures and techniques, analysis of clinical cases, theoretical and practical exercises, interactive summaries and master classes.
Delve into the latest advances in nanoparticles thanks to this innovative online teaching methodology, which allows you to decide when and where to study"
This Professional master’s degree in Biomedical Engineering contains the most complete and up-to-date scientific program on the market. The most important features include
- Case studies presented by experts in Biomedical Engineering
- The graphic, schematic, and practical contents with which they are created, provide scientific and practical information on the disciplines that are essential for professional practice
- Practical exercises where the self-assessment process can be carried out to improve learning
- Its special emphasis on innovative methodologies
- Theoretical lessons, questions to the expert, debate forums on controversial topics, and individual reflection assignments
- Content that is accessible from any fixed or portable device with an Internet connection
This program will provide you with an expert and highly experienced teaching staff, and numerous multimedia teaching resources with which you can quickly update your knowledge"
The program’s teaching staff includes professionals from the sector who contribute their work experience to this training program, as well as renowned specialists from leading societies and prestigious universities.
The multimedia content, developed with the latest educational technology, will provide the professional with situated and contextual learning, i.e., a simulated environment that will provide immersive training programmed to train in real situations.
This program is designed around Problem-Based Learning, whereby the professional must try to solve the different professional practice situations that arise during the academic year. For this purpose, the student will be assisted by an innovative interactive video system created by renowned and experienced experts.
With this Professional master’s degree, you will be able to incorporate the latest techniques in biomedical engineering into your professional practice.
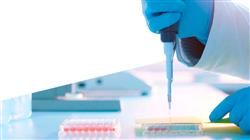
Get up to date with the latest scientific evidence on issues such as bio-devices or biomedical signals.
Syllabus
This Professional master’s degree in Biomedical Engineering is made up of 10 specialized modules in which the physician will be able to delve into the latest developments in stem cells, bionanomaterials, the different types of biomedical signals and the software to collect, measure and analyze them, the R programming language to perform statistical analysis of the data collected or nuclear medicine, among others.
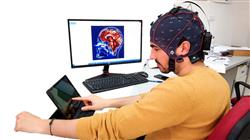
The most complete and up-to-date content in Biomedical Engineering is here"
Module 1. Tissue Engineering
1.1. Histology
1.1.1. Cellular Organization in Higher Structures: Tissues and Organs
1.1.2. Cellular Cycle Tissue Regeneration
1.1.3. Regulation: Interaction With the Extracellular Matrix
1.1.4. Importance of Histology in Tissue Engineering
1.2. Tissue Engineering
1.2.1. Tissue Engineering
1.2.2. Scaffolding
1.2.2.1. Properties
1.2.2.2. The Ideal Scaffolding
1.2.3. Biomaterials for Tissue Engineering
1.2.4. Bioactive Materials
1.2.5. Cells
1.3. Stem Cells
1.3.1. Stem Cells
1.3.1.1. Potentiality
1.3.1.2. Tests to Evaluate Potentiality
1.3.2. Regulation: Niche
1.3.3. Types of Stem Cells
1.3.3.1. Embryonic
1.3.3.2. IPS
1.3.3.3. Adult Stem Cells
1.4. Nanoparticles
1.4.1. Nanomedicine Nanoparticles
1.4.2. Types of Nanoparticles
1.4.3. Methods of Obtaining
1.4.4. Bionanomaterials in Tissue Engineering
1.5. Genetic Therapy
1.5.1. Genetic Therapy
1.5.2. Uses: Gene Supplementation, Cell Replacement, Cellular Reprogramming
1.5.3. Vectors for the Introduction of Genetic Material
1.5.3.1. Viral Vectors
1.6. Biomedical Applications of Tissue Engineering Products Regeneration, Grafts and Replacements
1.6.1. Cell Sheet Engineering
1.6.2. Cartilage Regeneration: Joint Repair
1.6.3. Corneal Regeneration
1.6.4. Skin Grafting for Major Burn Injuries
1.6.5. Oncology
1.6.6. Bone Replacement
1.7. Biomedical Applications of Tissue Engineering Products Circulatory, Respiratory and Reproductive System
1.7.1. Cardiac Tissue Engineering
1.7.2. Hepatic Tissue Engineering
1.7.3. Lung Tissue Engineering
1.7.4. Reproductive Organs and Tissue Engineering
1.8. Quality Control and Biosecurity
1.8.1. NCF Applied to Advanced Therapy Drugs
1.8.2. Quality Control
1.8.3. Aseptic Process: Viral and Microbiological Safety
1.8.4. Cell Production Unit: Characteristics and Design
1.9. Legislation and Regulation
1.9.1. Current Legislation
1.9.2. Authorization
1.9.3. Regulation of Advanced Therapies
1.10. Future Perspectives
1.10.1. Current Status of Tissue Engineering
1.10.2. Clinical Needs
1.10.3. Main Challenges at Present
1.10.4. Focus and Future Challenges
Module 2. Biomaterials in Biomedical Engineering
2.1. Biomaterials
2.1.1. Biomaterials
2.1.2. Types of Biomaterials and Application
2.1.3. Biomaterial Selection
2.2. Metallic Biomaterials
2.2.1. Types of Metallic Biomaterials
2.2.2. Properties and Current Challenges
2.2.3. Applications
2.3. Ceramic Biomaterials
2.3.1. Types of Ceramic Biomaterials
2.3.2. Properties and Current Challenges
2.3.3. Applications
2.4. Natural Polymeric Biomaterials
2.4.1. Interaction of Cells With Their Environment
2.4.2. Types of Biomaterials of Biological Origin
2.4.3. Applications
2.5. Synthetic Polymeric Biomaterials: In Vivo Behavior
2.5.1. Biological Response to Foreign Bodies (FBR)
2.5.2. In Vivo Behavior of Biomaterials
2.5.3. Biodegradation of Polymers Hydrolysis
2.5.3.1. Biodegradation Mechanisms
2.5.3.2. Degradation by Diffusion and Erosion
2.5.3.3. Hydrolysis Rate
2.5.4. Specific Applications
2.6. Synthetic Polymeric Biomaterials: Hydrogels
2.6.1. Hydrogels
2.6.2. Classification of Hydrogels
2.6.3. Hydrogel Properties
2.6.4. Hydrogel Synthesis
2.6.4.1. Physical Cross-Linking
2.6.4.2. Enzymatic Cross-Linking
2.6.4.3. Physical Cross-Linking
2.6.5. Structure and Swelling of Hydrogels
2.6.6. Specific Applications
2.7. Advanced Biomaterials: Intelligent Materials
2.7.1. Shape Memory Materials
2.7.2. Intelligent Hydrogels
2.7.2.1. Thermo-Responsive Hydrogels
2.7.2.2. PH Sensitive Hydrogels
2.7.2.3 Electrically Actuated Hydrogels
2.7.3. Electroactive Materials
2.8. Advanced Biomaterials: Nanomaterials
2.8.1. Properties
2.8.2. Biomedical Applications
2.8.2.1. Biomedical Images
2.8.2.2. Coatings
2.8.2.3. Focused Ligands
2.8.2.4. Stimulus-Sensitive Connections
2.8.2.5. Bio Markers
2.9. Specific Applications Neuroengineering
2.9.1. The Nervous System
2.9.2. New Approaches to Standard Biomaterials
2.9.2.1. Soft Biomaterials
2.9.2.2. Bioabsorbable Materials
2.9.2.3. Implantable Materials
2.9.3. Emerging Biomaterials Tissue Interaction
2.10. Specific Applications Biomedical Micromachines
2.10.1. Artificial Microswimmers
2.10.2. Contractile Microactuators
2.10.3. Small Scale Manipulation
2.10.4. Biological Machines
Module 3. Biomedical Signals
3.1. Biomedical Signals
3.1.1. Origin of Biomedical Signals
3.1.2. Biomedical Signals
3.1.2.1. Amplitude
3.1.2.2. Period
3.1.2.3. Frequency
3.1.2.4. Wavelength
3.1.2.5. Phase
3.1.3. Classification and Examples of Biomedical Signals
3.2. Types of Biomedical Signals Electrocardiography, Electroencephalography and Magnetoencephalography
3.2.1. Electrocardiography (ECG)
3.2.2. Electroencephalography (EEG)
3.2.3. Magnetoencephalography (MEG)
3.3. Types of Biomedical Signals Electroneurography and Electromyography
3.3.1. Electroneurography (ENG)
3.3.2. Electromyography (EMG)
3.3.3. Event-Related Potentials (ERPs)
3.3.4. Other Types
3.4. Signals and Systems
3.4.1. Signals and Systems
3.4.2. Continuous and Discrete Signals: Analog vs Digital
3.4.3. Systems in the Time Domain
3.4.4. Systems in Frequency Domain Spectral Method
3.5. Fundamentals of Signals and Systems
3.5.1. Sampling: Nyquist
3.5.2. The Fourier Transform DFT
3.5.3. Stochastic Processes
3.5.3.1. Deterministic Vs. Random Signals
3.5.3.2. Types of Stochastic Processes
3.5.3.3.Stationarity
3.5.3.4. Ergodicity
3.5.3.5. Relationships Between Signals
3.5.4. Power Spectral Density
3.6. Processing of Biomedical Signals
3.6.1. Processing of Signals
3.6.2. Objectives and Processing Steps
3.6.3. Key Elements of a Digital Processing System
3.6.4. Applications Tendencies
3.7. Filtering: Artifact Removal
3.7.1. Motivation Types of Filtering
3.7.2. Time Domain Filtering
3.7.3. Frequency Domain Filtering
3.7.4. Applications and Examples
3.8. Time-Frequency Analysis
3.8.1. Motivation
3.8.2. Time-Frequency Plane
3.8.3. Short Time Fourier Transform (STFT)
3.8.4. Wavelet Transform
3.8.5. Applications and Examples
3.9. Event Detection
3.9.1. Study Case I: ECG
3.9.2. Study Case II: EEG
3.9.3. Evaluation of Detection
3.10. Software for Biomedical Signal Processing
3.10.1. Applications, Environments and Programming Languages
3.10.2. Libraries and Tools
3.10.3. Practical Applications: Basic Biomedical Signal Processing System
Module 4. Biomechanics
4.1. Biomechanics
4.1.1. Biomechanics
4.1.2. Qualitative and Quantitative Analysis
4.2. Basic Mechanics
4.2.1. Functional Mechanisms
4.2.2. Basic Units
4.2.3. The Nine Fundamentals of Biomechanics
4.3. Mechanical Fundamentals Linear and Angular Kinematics
4.3.1. Linear Movement
4.3.2. Relative Movement
4.3.3. Angular Movement
4.4. Mechanical Fundamentals Linear Kinetics
4.4.1. Newton’s Law
4.4.2. Principle of Inertia
4.4.3. Energy and Work
4.4.4. Stress Angle Analysis
4.5. Mechanical Fundamentals Angular Kinetics
4.5.1. Torque
4.5.2. Angular Momentum
4.5.3. Newton's Angles
4.5.4. Balance and Gravity
4.6. Fluid Mechanics
4.6.1. Fluid
4.6.2. Flows
4.6.2.1. Laminar Flow
4.6.2.2. Turbulent Flow
4.6.2.3. Pressure-Velocity: The Venturi Effect
4.6.3. Forces in Fluids
4.7. Human Anatomy Limitations
4.7.1. Human Anatomy
4.7.2. Muscles: Active and Passive Stress
4.7.3. Mobility Range
4.7.4. Mobility-Strength Principles
4.7.5. Limitations in the Analysis
4.8. Mechanisms of the Motor System Bone, Muscle-Tendon and Ligament Mechanics
4.8.1. Tissue Functioning
4.8.2. Biomechanics of Bones
4.8.3.Biomechanics of the Muscle-Tendon Unit
4.8.4. Biomechanics of Ligaments
4.9. Mechanisms of the Motor System Mechanics of Muscles
4.9.1.Mechanical Characteristics of Muscles
4.9.1.1. Force-Speed Relationship
4.9.1.2. Force-Distance Relationship
4.9.1.3. Force-Time Relationship
4.9.1.4. Traction-Compression Cycles
4.9.1.5. Neuromuscular Control
4.9.1.6. The Spine and Backbone
4.10. Mechanics of Biofluids
4.10.1. Mechanics of Biofluids
4.10.1.1. Transport, Stress and Pressure
4.10.1.2. The Circulatory System
4.10.1.3. Blood Characteristics
4.10.2. General Problems in Biomechanics
4.10.2.1. Problems in Nonlinear Mechanical Systems
4.10.2.2. Problems in Biofluids
4.10.2.3. Solid-Liquid Problems
Module 5. Medical Bioinformatics
5.1. Medical Bioinformatics
5.1.1. Computing in Medical Biology
5.1.2. Medical Bioinformatics
5.1.2.1. Bioinformatic Applications
5.1.2.2. Computer Systems, Networks and Medical Databases
5.1.2.3. Applications of Medical Bioinformatics in Human Health
5.2. Computer Equipment and software Required in Bioinformatics
5.2.1. Scientific Computing in Biological Sciences
5.2.3. The Computer
5.2.4. Hardware, Software and Operating Systems
5.2.5. Workstations and Personal Computers
5.2.6. High-Performance Computing Platforms and Virtual Environments
5.2.7. Linux Operating System
5.2.7.1. Linux Installation
5.2.7.2. Using the Linux Command Line Interface
5.3. Data Analysis Using R Programming Language
5.3.1. Language R Statistical Programming
5.3.2. Installation and Uses of R
5.3.3. Data Analysis Methods With R
5.3.4. R Applications in Medical Bioinformatics
5.4. Data Analysis Using R Programming Language
5.4.1. Multipurpose Programming Language Python
5.4.2. Installation and Uses of Python
5.4.3. Data Analysis Methods With Python
5.4.4. Python Applications in Medical Bioinformatics
5.5. Methods of Human Genetic Sequence Analysis
5.5.1. Human Genetics
5.5.2. Techniques and Methods for Sequencing Analysis of Genomic Data
5.5.3. Sequence Alignments
5.5.4. Tools for Detection, Comparison and Modeling of Genomes
5.6. Data Mining in Bioinformatics
5.6.1. Phases of Knowledge Discovery in Databases, KDD
5.6.2. Processing Techniques
5.6.3. Knowledge Discovery in Biomedical Databases
5.6.4. Human Genomics Data Analysis
5.7. Artificial Intelligence and Big Data Techniques in Medical Bioinformatics
5.7.1. Machine Learning for Medical Bioinformatics
5.7.1.1. Supervised Learning Regression and Classification
5.7.1.2. Unsupervised Learning Clustering and Association Rules
5.7.2. Big Data
5.7.3. Computing Platforms and Development Environments
5.8. Applications of Bioinformatics for Prevention, Diagnosis and Clinical Therapies
5.8.1. Disease-Causing Gene Identification Procedures
5.8.2. Procedure to Analyze and Interpret the Genome for Medical Therapies
5.8.3. Procedures to Assess Genetic Predispositions of Patients for Prevention and Early Diagnosis
5.9. Medical Bioinformatics Workflow and Methodology
5.9.1. Creation of Workflows to Analyze Data
5.9.2. Application Programming Interfaces, APIs
5.9.2.1. R and Python Libraries for Bioinformatics Analysis
5.9.2.2. Bioconductor: Installation and Uses
5.9.3. Uses of Bioinformatics Workflows in Cloud Services
5.10. Factors Associated with Sustainable Bioinformatics Applications and Future Trends
5.10.1. Best Practices in the Development of Medical Bioinformatics Projects
5.10.2. Future Trends in Bioinformatics Applications
Module 6. Human-Machine Interface Applied to Biomedical Engineering
6.1. Human-Machine Interface
6.1.1. Human-Machine Interface
6.1.2. Model, System, User, Interface and Interaction
6.1.3. Interface, Interaction and Experience
6.2. Human-Machine Interaction
6.2.1. Human-Machine Interaction
6.2.2. Principles and Laws of Interaction Design
6.2.3. Human Factors
6.2.3.1. Importance of the Human Factor in the Interaction Process
6.2.3.2. Psychological-Cognitive Perspective: Information Processing, Cognitive Architecture, User Perception, Memory, Cognitive Ergonomics and Mental Models
6.2.4. Technological Factors
6.2.5. Basis of Interaction: Levels and Styles of Interaction
6.2.6. At the Forefront of Interaction
6.3. Interface Design (I): Design Process
6.3.1. Design Process
6.3.2 Value Proposition and Differentiation
6.3.3. Requirements Analysis and Briefing
6.3.4. Collection, Analysis and Interpretation of Information
6.3.5. The Importance of UX and UI in the Design Process
6.4. Interface Design (II): Prototyping and Evaluation
6.4.1. Prototyping and Evaluation of Interfaces
6.4.2. Methods for the Conceptual Design Process
6.4.3. Techniques for Idea Organization
6.4.4. Prototyping Tools and Process
6.4.5. Evaluation Methods
6.4.6. Evaluation Methods With Users: Interaction Diagrams, Modular Design, Heuristic Evaluation
6.4.7. Evaluation Methods Without Users: Surveys and Interviews, Card Sorting,A/B Testing and Design of Experiments
6.4.8. Applicable ISO Norms and Standards
6.5. User Interfaces (I): Interaction Methods in Current Technologies
6.5.1. User Interface (UI)
6.5.2. Classical User Interfaces: Graphical User Interfaces (GUIs), Web, Touch, Voice, etc.
6.5.3. Human Interfaces and Limitations: Visual, Hearing, Motor and Cognitive Diversity
6.5.4. Innovative User Interfaces: Virtual Reality, Augmented Reality, Collaborative
6.6. User Interfaces (II): Interaction Design
6.6.1. The Importance of Graphic Design
6.6.2. Design Theory
6.6.3. Design Rules: Morphological Elements, Wireframes, Use and Theory of Color, Graphic Design Techniques, Iconography, Typography
6.6.4 Semiotics Applied to Interfaces
6.7. User Experience (I): Methodologies and Design Fundamentals
6.7.1 User Experience (UX)
6.7.2 Evolution of Usability Effort-to-Benefit Ratio
6.7.3 Perception, Cognition and Communication
6.7.3.1 Mental Models
6.7.4. User Focused Design Methodology
6.7.5. Methodology of Design Thinking
6.8. User Experience (II): Principles of User Experience
6.8.1. UX Principles
6.8.2. UX Hierarchy: Strategy, Scope, Structure, Skeleton and Visual Component
6.8.3. Usability and Accessibility
6.8.4. Information Architecture: Classification, Labeling, Navigation, and Search Systems
6.8.5. Affordances and Signifiers
6.8.6 Heuristics: Heuristics of Understanding, Interaction and Feedback
6.9. Interfaces in the Field of Biomedicine (I): the Interaction of the Health Care Worker
6.9.1. Usability in the Intrahospital Context
6.9.2. Interaction Processes in Healthcare Technology
6.9.3. Health Care Provider and Patient Perception
6.9.4. Healthcare Ecosystem: Primary Care Physician Vs. Operating Room Surgeon
6.9.5. Interaction of the Healthcare Worker in a Context of Stress
6.9.5.1. ICU Cases
6.9.5.2. The Case of Extreme Circumstances and Emergencies
6.9.5.3. The Case of the Operating Rooms
6.9.6. Open Innovation
6.9.7. Persuasive Design
6.10. Interfaces in the Field of Biomedicine (II): Current Outlook and Future Trends
6.10.1. Classical Biomedical Interfaces in Healthcare Technologies
6.10.2. Innovative Biomedical Interfaces in Healthcare Technologies
6.10.3. The role of Nanomedicine
6.10.4. Biochips
6.10.5. Electronic Implants
6.10.6. Brain-Computer Interfaces (BCI)
Module 7. Biomedical Images
7.1. Biomedical Images
7.1.1. Medical Images
7.1.2. Objectives of Imaging Systems in Medicine
7.1.3. Types of Images
7.2. Radiology
7.2.1. Radiology
7.2.2. Conventional Radiology
7.2.3. Digital Radiology
7.3. Ultrasound
7.3.1. Medical Images With Ultrasound
7.3.2.Training and Image Quality
7.3.3. Doppler Ultrasound
7.3.4. Implementing and New Technologies
7.4. Computerized Tomography
7.4.1. CT Imaging Systems
7.4.2. Reconstruction and CT Image Quality
7.4.3. Clinical Applications
7.5. Magnetic Resonance
7.5.1. Magnetic Resonance Imaging (MRI)
7.5.2.Resonance and Nuclear Magnetic Resonance
7.5.3. Nuclear Relaxation
7.5.4. Tissue Contrast and Clinical Applications
7.6. Nuclear Medicine
7.6.1. Generation and Image Detection
7.6.2. Image Quality
7.6.3. Clinical Applications
7.7. Image Processing
7.7.1. Noise
7.7.2. Intensification
7.7.3. Histograms
7.7.4. Magnification
7.7.5. Processing
7.8. Analysis and Image Segmentation
7.8.1. Segmentation
7.8.2. Segmentation by Region
7.8.3. Edge Detection Segmentation
7.8.4. Generation of Biomodels From Images
7.9. Image-Guided Interventions
7.9.1. Visualization Methods
7.9.2. Image-Guided Surgeries
7.9.2.1. Planning and Simulation
7.9.2.2. Surgical Visualization
7.9.2.3. Virtual Reality
7.9.3. Robotic Vision
7.10. Deep Learning and Machine Learning in Medical Imaging
7.10.1. Types of Recognition
7.10.2. Supervised Techniques
7.10.3. Unsupervised Techniques
Module 8. Digital Health Applications in Biomedical Engineering
8.1. Digital Health Applications
8.1.1. Medical Hardware and Software Applications
8.1.2. Software Applications: Digital Health Systems
8.1.3. Usability of Digital Health Systems
8.2. Medical Image Storage and Transmission Systems
8.2.1. Image Transmission Protocol: DICOM
8.2.2. Medical Image Storage and Transmission Server Installation: PAC System
8.3. Relational Database Management for Digital Health Applications
8.3.1. Relational Database, Concept and Examples
8.3.2. Database Language
8.3.3. Database With MySQL and PostgreSQL
8.3.4. Applications: Connection and Uses in Web Programming Language
8.4. Digital Health Applications Based on Web Development
8.4.1. Web Application Development
8.4.2. Web Development Model, Infrastructure, Programming Languages and Working Environments
8.4.3. Examples of Web Applications With the Languages: PHP, HTML, AJAX, CSS Javascript, AngularJS, NodeJS
8.4.4. Development of Applications in Web Frameworks: Symfony and Laravel
8.4.5. Development of Applications in Content Management Systems, CMS: Joomla and WordPress
8.5. WEB Applications in a Hospital Environment or Clinical Center
8.5.1. Applications for Patient Management: Reception, Scheduling and Collections
8.5.2. Applications for Medical Professionals Consultations or Medical Care, Medical History, Medical Reports, Medical Records
8.5.3. Web and Mobile Applications for Patients: Agenda Requests, Monitoring
8.6. Telemedicine Applications
8.6.1. Service Architecture Models
8.6.2. Telemedicine Applications: Teleradiology, Teleradiology, Telecardiology and Teledermatology
8.6.3. Rural Telemedicine
8.7. Applications With the Internet of Medical Things, IoMT
8.7.1. Models and Architectures
8.7.2. Medical Data Acquisition Equipment and Protocols
8.7.3. Applications: Patient Monitoring
8.8. Digital Health Applications Using Artificial Intelligence Techniques
8.8.1. Machine Learning
8.8.2. Computing Platforms and Development Environments
8.8.3. Examples
8.9. Digital Health Applications with Big Data
8.9.1. Digital Health Applications with Big Data
8.9.2. Technologies Used in Big Data
8.9.3. Use Cases of Big Data in Digital Health
8.10. Factors Associated With Sustainable Digital Health Applications and Future Trends
8.10.1. Legal and Regulatory Framework
8.10.2. Best Practices in the Development of Digital Health Application Projects
8.10.3. Future Trends in Digital Health Applications
Module 9. Biomedical Technologies: Biodevices and Biosensors
9.1. Medical Devices
9.1.1. Product Development Methodology
9.1.2. Innovation and creativity
9.1.3. CAD Technologies
9.2. Nanotechnology
9.2.1. Medical Nanotechnology
9.2.2. Nanostructured Materials
9.2.3. Nano-Biomedical Engineering
9.3. Micro and Nanofabrication
9.3.1. Design of Micro and Nano Products
9.3.2. Techniques
9.3.3. Tools for Manufacturing
9.4. Prototypes
9.4.1. Additive Manufacturing
9.4.2. Rapid Prototyping
9.4.3. Classification
9.4.4. Applications
9.4.5. Study Cases
9.4.6. Conclusions
9.5. Diagnostic and Surgical Devices
9.5.1. Development of Diagnostic Methods
9.5.2. Surgical Planning
9.5.3. Biomodels and Instruments Made With 3D Printing
9.5.4. Device-Assisted Surgery
9.6. Biomechanic Devices
9.6.1. Prosthetists
9.6.2. Intelligent Materials
9.6.3. Orthotics
9.7. Biosensors
9.7.1. Biosensor
9.7.2. Sensing and Transduction
9.7.3. Medical Instrumentation for Biosensors
9.8. Typology of Biosensors (I): Optic Sensors
9.8.1. Reflectometry
9.8.2. Interferometry and Polarimetry
9.8.3. Evanescent Field
9.8.4. Fiber Optic Probes and Guides
9.9. Typology of Biosensors (II): Physical, Electrochemical and Acoustic Sensors
9.9.1. Physical Sensors
9.9.2. Electrochemical Sensors
9.9.3. Acoustic Sensors
9.10. Integrated Systems
9.10.1. Lab-On-A-Chip
9.10.2. Microfluidics
9.10.3. Medical Application
Module 10. Biomedical and Healthcare Databases
10.1. Hospital Databases
10.1.1. Data Bases
10.1.2. The Importance of Data
10.1.3. Data in a Clinical Context
10.2. Conceptual Modeling
10.2.1. Data Structure
10.2.2. Systematic Data Model
10.2.3. Data Standardization
10.3. Relational Data Model
10.3.1. Advantages and Disadvantages
10.3.2. Formal Languages
10.4. Designing from Relational Databases
10.4.1. Functional Dependence
10.4.2. Relational Forms
10.4.3. Standardization
10.5. SQL Language
10.5.1. Relational Model
10.5.2. Object-Relationship Model
10.5.3. XML- Object-Relationship Model
10.6. NoSQL
10.6.1. JSON
10.6.2. NoSQL
10.6.3. Differential Amplifiers
10.6.4. Integrators and Differentiators
10.7. MongoDB
10.7.1. ODMS Architecture
10.7.2. NodeJS
10.7.3. Mongoose
10.7.4. Aggregation
10.8. Data Analysis
10.8.1. Data Analysis
10.8.2. Qualitative Analysis
10.8.3. Quantitative Analysis
10.9. Legal Bases and Regulatory Standards
10.9.1. General Data Protection Regulation
10.9.2. Cybersecurity Considerations
10.9.3. Regulations Applied to Health Data
10.10. Integration of Databases in Medical Records
10.10.1. Medical History
10.10.2. HIS Systems
10.10.3. HIS Data
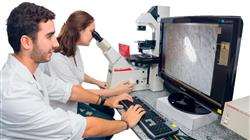
In this Professional master’s degree you will have a teaching staff of excellence, the most updated contents in the discipline and a teaching methodology that will allow you to combine your studies with your professional career"
Professional Master's Degree in Biomedical Engineering
Biomedical engineering is a discipline that combines engineering and biology to create technological solutions to medical and health problems. This discipline focuses on applying engineering in the research, design, development and maintenance of medical equipment, devices and systems, such as prostheses, medical diagnostic equipment, therapy and rehabilitation equipment, among others.
This branch of engineering uses principles from different areas, such as mechanics, electronics, computer science, physics and chemistry, to create solutions for health and wellness problems. Biomedical engineering focuses on several areas, such as medical imaging, biomechanics, tissue engineering, bioinformatics, health systems engineering, and ergonomics, among others. Biomedical engineers work closely with physicians, scientists and technology experts to create innovative solutions that can improve patients' quality of life and assist healthcare professionals in their daily work.
Biomedical engineering focuses on many fields, including biomechanics, biocompatible materials engineering, electrical and electronics engineering, software engineering, biomedical imaging engineering and medical systems engineering.
Biomedical engineers are highly sought after by the medical industry, universities and research centers. In addition, they are recognized for their ability to develop creative and efficient solutions to medical and healthcare challenges.
TECH the world's largest onlinel university has a specialized Professional Master's Degree designed to provide advanced knowledge and technical skills in the field of biomedical engineering.
The objective of the Professional Master's Degree is to provide a solid understanding of biomedical engineering and its application in the design and development of biomedical devices and systems. They will learn the most advanced techniques and the most effective strategies for engineering biomedical solutions. The Professional Master's Degree is intended for graduates of engineering, life sciences and related disciplines who wish to specialize in biomedical engineering, as well as those working in the biomedical industry and research and development organizations.